Heart changes in newborns.
The article:
Breckenridge RA, et al. (2013) Hypoxic Regulation of Hand1 Controls the Fetal-Neonatal Switch in Cardiac Metabolism. PLoS Biol 11(9): e1001666. doi:10.1371/journal.pbio.1001666
Subject areas: Developmental Biology
Vocabulary:
heart attack – When a blood vessel supplying oxygen-rich blood to the heart is blocked, part of the heart is deprived of oxygen, which causes it to stop functioning and the cells in that part of the heart will soon begin dying. In medical terminology, it is also called a myocardial infarction.
ischemia – restriction of blood to a tissue/organ, often causing damage due to lack of oxygen.
—–
The amount of work that a fetal heart must do, and the environment in which it does that work, is very different from the work that it must do once it has been born. This article explores the mechanism behind the giant shift that occurs in the most basic level of how the heart works as it goes from the relatively low-oxygen environment inside the womb to the high-oxygen environment of our atmosphere once it is born.
ATP, or adenosine triphosphate, is the energy currency of most life. It is a small molecule that temporarily stores energy in some of its chemical bonds. That energy can be released by the cells when needed to power the many processes that keep a cell alive. ATP, however, is not a molecule that is just floating around for cells to take up and use. While it is great at holding energy temporarily for quick use, there are much more efficient ways of storing large amounts of energy – mostly in large macromolecules like fats or glycogen. In most animal cells, there are two basic ways that ATP can be made. There is glycolysis and there is oxidative phosphorylation.
Glycolysis only produces 2 ATP from a single glucose molecule (the simple sugar glucose is the basic “food” of the cell). That is enough to maintain a low level of activity in the cell, but not much beyond that. In comparison, oxidative phosphorylation can produce nearly 40 ATP per glucose molecule. On the surface, one might question why glycolysis would ever be used. The key distinction is that glycolysis does not require oxygen. So, when oxygen is not plentiful, glycolysis is a more reliable and steady source of ATP.
Now it kind of makes sense, right? Inside the womb where there is relatively low oxygen, the fetal heart has a primarily glycolytic metabolism, but once it is born and its lungs are breathing in the oxygen-rich atmosphere, it makes use of the aerobic pathway to generate more ATP quickly for a heart that is also having to work harder. In the article, the authors refer to a switch from glycolysis to lipid oxidation. Lipid oxidation is just breaking down of fats into chemical products that eventually enter into the oxidative phosphorylation process.
For more detail on these metabolic processes, you can read Chapter 5 in our free textbook, Cells: Molecules and Mechanisms.
But back to the article…
What they knew.
In mice, the switch from glycolysis to lipid oxidation happens very quickly, within a week of birth. Other scientists had previously discovered a transcription factor, Hand1, which is a crucial regulator of early embryogenesis, and particularly of early heart development. The authors came to Hand1 from almost the opposite direction, having found it to be involved in aging, failing heart muscle. Because failing heart muscle appears to undergo metabolic changes as well, they wondered if Hand1 could regulate both the developmental switch and the metabolic changes in failing heart cells.
What (and how) they tested.
In order to show involvement, the authors would want to re-confirm the expression of Hand1 in the fetal heart up until birth. This should then change in some way during the week after birth, coinciding with the metabolic change in the newborn heart cells. They showed this by dissecting the heart from mice pups of ages e18.5 (embryonic day 18.5, still in the womb but about to be born) through p6.5 (postnatal day 6.5, about 6.5 days after birth), and extracting the mRNA. They probed the total mRNA for Hand1, and as you can see in figure A below, the amount of Hand1 mRNA drops off dramatically after birth. In contrast, the amount of mRNA for Hand2, a related gene, does not change at all (figure B).
Knowing that Hand1 is changing levels at the right time, next the research team wanted to demonstrate that Hand1 is actually the determining factor, and not just coincidentally being downregulated. Since it is normally downregulated, they decided to see what would happen if it did not go down, but continued to be produced at the same level as before birth. To do this, they created transgenic mice with an inducible Hand1 gene. Basically, what this means is that when they introduce doxycycline (a commonly used antibiotic that does not have any known effect on heart development) to the animal, Hand1 will be produced.
This way, they don’t change the natural regulation of Hand1 before birth, but shortly before the mother is expected to give birth, she is injected with doxycycline, which travels through her bloodstream and into her fetal pups. This activates the extra Hand1 gene in them, so that it continues to be produced even after birth. Checking for Hand1 mRNA in the heart like before, they found that Hand1 was present after birth at significantly higher levels than normal, and about 60% of the pre-birth level in normal fetal mice (figure below, left).

(A) Hand1 mRNA expression just prior to and after birth. Blue columns are control mice, black columns are transgenic Hand1 overexpressing mice. (B) c is control mouse, oe is Hand1-overexpressing.
The mice that expressed Hand1 after birth were externally normal at first glance (figure above, right). However, closer examination showed that the mice were cyanotic (having a bluish color from lack of oxygen to blood), and the heart mass was significantly lower in the induced Hand1 mice than in normal mice. Furthermore, neonatal death rates were higher for the induced mice, and a rupture of the left ventricle was observed in some of the dead mice.
They did find though, that there was a significantly lower ratio of glycogen to glucose in the Hand1-induced mice than in the controls, indicating that they were metabolizing by glycolysis much more than normal mice (which at that point would be using less glycogen, more fatty acids). In fact, checking the expression of a number of genes that encode enzymes used in lipid metabolism, the figure below shows very clearly that many, are significantly changed in the induced Hand1 neonate.
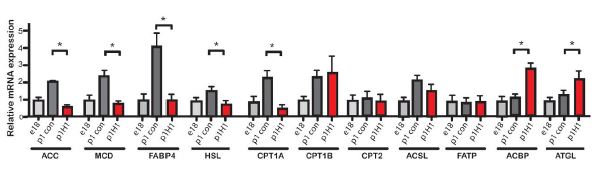
Expression levels of various genes involved in lipid metabolism. Red columns are from Hand1-expressing postnatal hearts. * marks statistically significant differences.
What does that show?
It shows that in normal mice, the downregulation of Hand1 is necessary for the switch from a primarily anaerobic to a primarily aerobic metabolism, and this needs to happen around the time of birth.
But wait, there’s more.
What triggers the downregulation? Is it timing? Or could it be exposure to oxygen? The figure at right shows what happens to Hand1 expression when adult mice are kept in a low-oxygen environment for two weeks. Hypoxic conditions increased the expression of Hand1 significantly, even though the adult mice normally do not express it. As it turns out, this falls in line with the authors’ previous work on heart muscle in failing hearts.
During a heart attack or even during heart surgery, the heart muscle is very susceptible to damage from hypoxic conditions. It has been known that as the heart is failing (e.g. due to heart attack), the cells in the heart begin to express enzymes and proteins that are more characteristic of fetal heart than adult heart. This could be a way for the heart cells to protect themselves and try to stay alive under low-oxygen conditions.
So Hand1 is expressed (or technically, re-expressed) in adult heart cells under low oxygen conditions. Does it actually help to deal with the low oxygen? The figure at left shows an experiment done on a culture of heart muscle cells. When the cells were transfected so that they would express Hand1, the basal oxygen consumption, the maximum respiration, the ATP production, and the spare (unused) respiratory capacity all fell significantly.
Finally, there is an interesting result that still needs to be explored further before people get excited about it as a medical advance. Using a model system in which they modeled ischemia by perfusing adult mouse hearts in a hypoxic buffer for 35 minutes before replacing it with normal-oxygen media. As you can see in the graph at right, the heart that was expressing Hand1 had a 47% reduction in heart tissue damage!
No comments
Be the first one to leave a comment.